Effects of bacterial β-mannanase on apparent total tract digestibility of nutrients in various feedstuffs fed to growing pigs
Article information
Abstract
Objective
The objective of this study was to determine the effects of β-mannanase on metabolizable energy (ME) and apparent total tract digestibility (ATTD) of protein in various feedstuffs including barley, copra meal, corn, corn distillers dried grains with solubles (DDGS), palm kernel meal, sorghum, and soybean meal.
Methods
A basal diet was formulated with 94.8% corn and 0.77% amino acids, minerals, and vitamins and test diets replacing corn-basal diets with barley, corn DDGS, sorghum, soybean meal, or wheat (50%, respectively) and copra meal or palm kernel meal (30%, respectively). The basal diet and test diets were evaluated by using triplicated or quadruplicated 2×2 Latin square designs consisting of 2 diets and 2 periods with a total of 54 barrows at 20.6±0.6 kg (9 wk of age). Dietary treatments were levels of β-mannanase supplementation (0 or 800 U/kg of feed). Fecal and urine samples were collected for 4 d following a 4-d adaptation period. The ME and ATTD of crude protein (CP) in feedstuffs were calculated by a difference procedure. Data were analyzed using Proc general linear model of SAS.
Results
Supplementation of β-mannanase improved (p<0.05) ME of barley (10.4%), palm kernel meal (12.4%), sorghum (6.0%), and soybean meal (2.9%) fed to growing pigs. Supplementation of β-mannanase increased (p<0.05) ATTD of CP in palm kernel meal (8.8%) and tended to increase (p = 0.061) ATTD of CP in copra meal (18.0%) fed to growing pigs.
Conclusion
This study indicates that various factors such as the structure and the amount of β-mannans, water binding capacity, and the level of resistant starch vary among feedstuffs and the efficacy of supplemental β-mannanase may be influenced by these factors.
INTRODUCTION
Barley, corn, sorghum, soybean meal, and wheat are commonly used energy feeds and protein supplements in pig feeds. Due to the volatility of their economical values, some other alternative feedstuffs have been considered in feeding pigs including corn distillers dried grains with solubles (DDGS), copra meal, or palm kernel meal depending on availability in different regions [1–3]. Some of conventional and alternative feedstuffs contain high levels of non-starch polysaccharides (NSP), which are not hydrolyzed by digestive enzymes and act as anti-nutritional factors causing negative impacts on nutrient utilization in pigs [4–6]. Exogenous microbial enzymes have been considered as feed additives to improve the nutrient utilization of pigs fed with alternative feedstuffs [7–9].
Beta-mannans are complex polysaccharides that are commonly found in plant cell walls. According to Kiarie et al [10], copra meal (32.6% of total β-mannans), palm kernel meal (30.9%), and soybean meal (1.7%) are considered high sources of β-mannans compared with other feedstuffs, including barley (0.4%), corn (0.3%), corn DDGS (1.3%), sorghum (0.1%), and wheat (0.3%). Beta-mannans can also be classified in four subfamilies: linear mannan, glucomannan, galactomannan, and galactoglucomannan based on various molecules bound to β-mannan backbone such as galactose or glucose [11]. The backbone of mannan or β-glucomannan can be substituted with side chains of α-1,6-linked galactose or glucose residues. In particular, soluble β-mannans could have high viscous property and negative impacts on nutrient absorption of pigs [10]. The solubility of the β-mannans could be determined by muliple factors; i) the degree of branching, which has a positive correlation with solubility due to increased hydrophilicity with more branching, ii) the length and composition of the side chains, which can either positively or negatively impact solubility depending on their hydrophilic or hydrophobic characteristics, and iii) molecular weight, which typically has a negative correlation with solubility [12]. Beta-mannans with simple backbone structures or short side chains of hydrophobic sugars (glucose or mannose) cause increased viscosity and interfered nutrient utilization in the intestine of pigs. Although there is limited information on the characterization of specific β-mannans in the feedstuffs, the difference in β-mannan profiles among feedstuffs may cause variations in the extent of the impact on the performance and health of pigs.
Beta-mannanase has been used to hydrolyze mannans in pig feeds to improve nutrient utilization by reducing digesta viscosity, releasing nutrients for digestion [13,14], and releasing mannan-oligosaccharides which can act as prebiotics supporting the intestinal health of pigs [10,15,16]. In turn, β-mannanase could improve nutrient utilization and finally growth of pigs [10]. Efficacy of feed enzymes could vary depending on the composition of feeds due to differences in amount and structure of targeting substrates among feedstuffs [17]. It is necessary to investigate the effects of a feed enzyme on each of specific feedstuffs, which allows the estimation of its impact on feeds composed of these feedstuffs.
It is hypothesized that energy utilization and protein digestibility in various feedstuffs can be improved by the use of β-mannanase in feeds for pigs, whereas the extent of improvement can vary depending on feedstuffs composing the feed. The objective of this study was to determine the effects of β-mannanase on metabolizable energy (ME) and apparent total tract digestibility (ATTD) of protein in selected major feedstuffs including barley, copra meal, corn, corn DDGS, palm kernel meal, sorghum, and soybean meal.
MATERIALS AND METHODS
Animal care
The experimental protocol was approved by the Institutional Animal Care and Use Committee at North Carolina State University. The experiment was conducted at the Swine Educational Unit at North Carolina State University (Raleigh, NC, USA).
Diets, animal, and experimental design
A basal diet was formulated with corn (94.8%) and supplemental amino acids to provide energy and essential amino acids (Table 1). Seven additional test diets were formulated by replacing a portion of the corn-basal diet with barley, corn DDGS, sorghum, soybean meal, or wheat at 50%, or with copra meal or palm kernel meal at 30%. The basal diet and test diets were evaluated by using triplicated or quadruplicated 2×2 Latin square designs consisting of 2 diets and 2 periods using a total of 54 barrows at 20.6±0.6 kg (9 wk of age). Dietary treatments were levels of β-mannanase supplementation (0 or 800 U/kg of feed). Triplicated 2×2 Latin square design was used for copra meal, corn DDGS, palm kernel meal, sorghum, and wheat with or without β-mannanase. Quadruplicated 2×2 Latin square design was used for barley, corn, and soybean meal with or without β-mannanase. Beta-mannanase (800,000 U/kg; CTCBIO Inc., Seoul, Korea) was produced from the fermentation by Bacillus subtilis isolate WL-7 (GenBank no. AAT27435.1) on Luria broth. The analyzed compositions of energy and nutrient composition of the feedstuffs used in this study are shown in Table 2.
Experimental procedures and analysis
Pigs received a fixed amount of experimental diets twice daily (0800 and 1700 h) based on body weight (BW) of pigs (daily feed allowance = 0.09×BW0.75 kg). Pigs were weighed at the end of each period to adjust feed allowance for a subsequent period. Daily feed intake were recorded considering any feed refusal. For each phase, on d 4 at 1700 h, chromic oxide (0.3%) was added to the evening meal as an external marker to indicate the initiation of fecal collection. Sampling was done for 4-d consecutively. Fecal collection was initiated when green color from chromic oxide was visually observed in the feces after feeding a meal with chromic oxide as an indigestible maker, whereas urine sampling was initiated after the time of feeding a meal with chromic oxide. On d 8 1700 h, chromium oxide at 0.5% was added to the evening meal as an external marker for fecal collection. Fecal sampling was terminated when green color was observed in the feces in the following morning. Urine collection was terminated at the time of evening meal on d 8. Urine was collected in a plastic container with 20 mL HCl (6 N). The volume of urine was measured each day during the collection period, and 150 mL of the urine sample was subsampled daily. Fecal samples were weighed at the end of each day during measurement period. Urine and fecal samples were frozen (−20°C) immediately after collection.
The frozen fecal and urine samples were dried in a forced-air oven at 65°C. Daily collection of urine samples from each pig were pooled proportionally depending on the volume of urine collected on each day. Feed, urine, and fecal samples were analyzed for gross energy (GE) and crude protein (CP). Gross energy was obtained using a bomb calorimeter (Model 6300; Parr Instruments, Moline, IL, USA). Crude protein was obtained with the combustion method (method 999.03; AOAC International, 2007).
Calculation and statistical analysis
Energy values from the excretion of GE in the feces and urine were subtracted from the intake of GE to calculate digestible energy (DE) and ME for each diet [18]. The DE and ME in barley, copra meal, corn DDGS, palm kernel meal, sorghum, soybean meal, or wheat were then calculated by a difference procedure [18,19]. The ATTD of CP was also calculated for each feedstuff by a difference procedure [18,19].
For each experiment, data were analyzed using Proc general linear model of SAS (SAS Inc., Cary, NC, USA). The experiment was based on a Latin square design, and the experimental unit was the individual pig. Period and Latin square were included as fixed effects, and pig was included as a random effect. The experimental unit was the individual pig. The statistical difference was considered significant with p<0.05, whereas 0.05≤p<0.10 was considered as tendency.
RESULTS
Supplementation of β-mannanase did not affect GE intake in pigs fed diets containing barley, corn, corn DDGS, sorghum, or wheat (Table 3). Supplementation of β-mannanase did not affect fecal excretion of GE in pigs fed diets containing corn, corn DDGS, or wheat. Fecal excretions of GE in pigs fed diets containing barley or sorghum were reduced (p<0.05) by supplementation of β-mannanase. Supplementation of β-mannanase did not affect ATTD of GE in pigs fed diets containing corn, corn DDGS, or wheat. The ATTD of GE in the pigs fed diets containing barley or sorghum were increased (p<0.05) by supplementation of β-mannanase. Supplementation of β-mannanase did not affect DE and ME values of diets containing corn, corn DDGS, or wheat. The DE and ME values of diets containing barley were increased (p<0.05) by supplementation of β-mannanase. The DE value of diets containing sorghum tended to be increased (p = 0.052) and the ME value of the diet containing sorghum was also increased (p<0.05) by supplementation of β-mannanase.
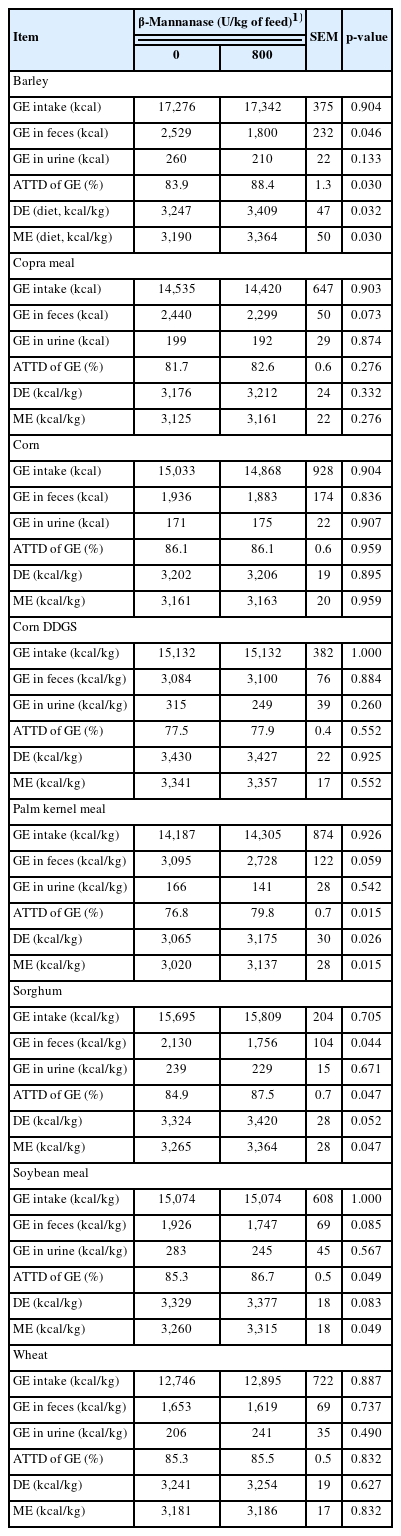
Energy balance (dry matter basis) in feeds with various feedstuffs fed to growing pigs with or without β-mannanase
Supplementation of β-mannanase did not affect GE intake of pigs fed diets containing copra meal, palm kernel meal, or soybean meal. Fecal excretions of GE in pigs fed diets containing copra meal (p = 0.073), palm kernel meal (p = 0.059) or soybean meal (p = 0.085) tended to be reduced by supplementation of β-mannanase. Supplementation of β-mannanase did not affect ATTD of GE in pigs fed diets containing copra meal. The ATTD of GE in the pigs fed diets containing palm kernel meal or soybean meal were increased (p<0.05) by supplementation of β-mannanase. Supplementation of β-mannanase did not affect DE and ME values of diets containing copra meal. The DE and ME values of diets containing palm kernel meal were increased (p<0.05) by supplementation of β-mannanase. The DE value of diets containing sorghum tended to be increased (p = 0.052) and the ME value of the diet was increased (p<0.05) by supplementation of β-mannanase.
Supplementation of β-mannanase did not affect CP intake and fecal excretion of CP in pigs fed diets containing barley, copra meal, corn, corn DDGS, palm kernel meal, sorghum, soybean meal, or wheat (Table 4). Supplementation of β-mannanase did not affect ATTD of CP in pigs fed diets containing barley, corn, corn DDGS, sorghum, soybean meal, or wheat. The ATTD of CP in the pigs fed diets containing copra meal tended to be increased (p = 0.061) by supplementation of β-mannanase. The ATTD of CP in the pigs fed diets containing palm kernel meal was increased (p<0.05) by supplementation of β-mannanase.
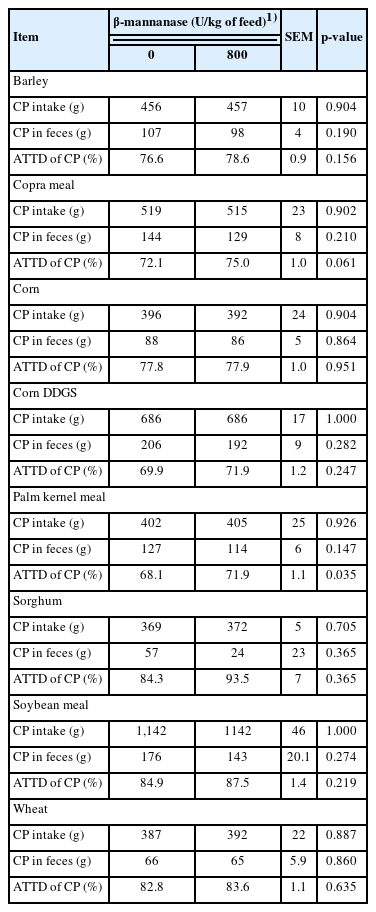
Apparent total tract digestibility (ATTD) of crude protein (CP) in feeds with various feedstuffs fed to growing pigs with or without β-mannanase
Supplementation of β-mannanase did not affect ME and ATTD of CP in corn, corn DDGS, and wheat fed to growing pigs (Table 5). Supplementation of β-mannanase improved (p<0.05) ME in barley, palm kernel meal, sorghum, and soybean meal fed to growing pigs. Supplementation of β-mannanase increased (p<0.05) ATTD of CP in palm kernel meal and tended to increase (p = 0.061) ATTD of CP in copra meal fed to growing pigs.
DISCUSSION
Beta-mannanase is an enzyme that hydrolyzes β-mannans, complex polysaccharides in plant-based feedstuffs commonly used in swine diets, such as soybean meal, copra meal, and palm kernel meal. The addition of β-mannanase to swine feed has been shown to have several beneficial effects, including improved growth performance, nutrient digestibility, and enhanced immunity [15,20]. Supplementation of β-mannanase has been associated with reducing the negative impacts of β-mannans, which are anti-nutritional factors that can bind to nutrients and reduce its digestibility, leading to reduced growth performance in pigs [10]. It has been also known that β-mannanase breaks down these complex polysaccharides having cage effects, which physically interfere with the mode of action of endogenous or microbial enzymes to hydrolyze the nutrients inside the cell wall [21,22]. This study also shows that supplementation of β-mannanase could improve ME in barley, palm kernel meal, sorghum, and soybean meal as well as protein digestibility in copra meal and palm kernel meal. These improvement could be related to the DE of feeds containing these feedstuffs increased by supplementation of β-mannanase without affecting urinary energy. This could suggest that the β-mannanase make the nutrients more accessible for absorption, thereby contributing to an increase in ME in barley, palm kernel meal, sorghum, and soybean meal. This study can indicate the potential of β-mannanase supplementation in improving the energy utilization from barley, palm kernel meal, sorghum, and soybean meal by enhancing nutrient digestibility. Therefore, the nutrient contributions of these feedstuffs in swine feeds should be reconsidered when β-mannanase is supplemented.
In this study, the compositions of analyzed GE and CP in feedstuffs were similar except copra meal and palm kernel meal compared with the values described in NRC [23]. Comparing with previous studies, the CP in copra meal (25.7%) is slightly higher than the values (21.1%±0.7%) based on the previous reports [24–27], although the GE and CP in palm kernel meal are within the range of the values from previous studies [28–30]. According to Stein et al [31], copra meal could have variations in the nutrient composition mainly due to a differences in residual oil contents from expeller or solvent extraction.
The β-mannanase is an enzyme that breaks down β-mannans, which are contained at various levels depending on the feedstuffs [10]. Interestingly, this study shows that ME in corn, corn DDGS, wheat, or copra meal were not affected whereas ME of barley, sorghum, palm kernel meal, or soybean meal increased when β-mannanase was supplemented. The results could also indicate that the effects of β-mannanase on the ME in feedstuffs did not correspond with the β-mannan content or the ratio of soluble to total β-mannans within these feedstuffs (Table 6). Several potential reasons for these results can be speculated. The NSP compounds interact with other components in the cell wall structure of the feedstuffs. In the plant cell wall, β-mannans are typically present in the middle lamella and primary cell wall, where β-mannans contribute to the structure and rigidity [32,33]. The β-mannans interact with other polysaccharides and nutrients to form a complex that provides the cell with strength and flexibility [34–36]. If β-mannan molecules are surrounded by other NSP components, it would limit β-mannanase to physically access them, interfering with the enzymatic reaction [37,38]. Thus, the efficacy to β-mannanase would depend on physical structure of β-mannans in relation to other NSP components. In addition, the types of link and degrees of polymerization structuring β-mannans in each feedstuff vary greatly, whereas there are no clear understanding how these can influence the interaction with β-mannanase warranting further research [39].
Copra meal is rich in β-mannans, and also contains greater amounts of fat and protein as well as soluble fiber, but lower energy value compared with palm kernel meal [2]. According to Jaworski et al [40], the water binding capacity of copra meal was greater than of palm kernel meal. Water binding capacity refers to the ability of feedstuffs to hold the moisture, which is an important factor in the processing and digesting feedstuffs [41]. Soluble fiber with higher water binding capacity tend to form a viscous substance that can trap nutrients and enzymes, making them less accessible for nutrient digestion [42,43]. Feedstuffs with high water binding capacity such like copra meal can make it more difficult for digestive or exogenous enzymes to access and break down the nutrients entrapped. Previous studies showed that energy digestion of growing pigs did not response to supplementation of β-mannanase when they fed with copra meal [2,44]. This study shows that ATTD of CP in pigs fed diets containing copra meal or palm kernel meal was increased by supplementation of β-mannanase. Soluble β-mannans possessing high water binding capacity may negatively affect the protein utilization due to the hydrophilic characteristic in protein. Therefore, the results in this study indicate that there are needs to consider both the water binding capacity of feedstuffs and the enzyme activity when formulating swine feeds with exogenous enzymes.
This study shows that the ME of barley and sorghum containing relatively low levels of β-mannans was increased by the supplementation of β-mannanase. Among the feedstuffs with low NSP, Navarro et al [45] showed that barley (5.5%) and sorghum (16.2%) contain significant greater resistant starch compared with corn (1.0%) and wheat (0.4%). Resistant starch is a type of starch that is resistant to digestion in the small intestine and travels to the large intestine, where it acts as a prebiotic to promote the growth of beneficial intestinal bacteria [46]. The β-mannanase can help breaking down the NSP structure, making the resistant starch more accessible for digestion and absorption in the small intestine [47].
In addition, the ME of soybean meal was also increased by supplementation of β-mannanase. Soybean meal contains relatively high β-mannans compared with other feedstuffs such as barley, corn DDGS, corn, sorghum, and wheat [10]. Specifically, NSP can interfere the interactions among lipase, fat, and bile salt micelles in the digestive tract, ultimately leading to reduced fat digestion [48]. The study suggests that the improved energy digestibility may be due to the breakdown of β-mannans, which are primarily galactomannans, a type of soluble NSP composed of 1,4-linked β-D-mannopyranosyl residues in soybean meal [10,49,50]. The composition or types of β-mannans present in feedstuffs may influence their interactions with other nutrients or the substrate effects, potentially leading to varying responses to β-mannanase. However, supplementation of β-mannanase did not affect ATTD of CP of the pigs fed with soybean meal. One possible reason would be related to that the protein in soybean meal highly digestible for growing pigs. The results also show that soybean meal has the highest protein digestibility among the other feedstuffs. Soy protein is generally considered to be highly digestible for pigs, with a protein digestibility value of around 80% or higher [51]. Moreover, this study also shows that the changes in the ATTD of CP by supplementation of β-mannanase were detected in copra meal and palm kernel showing the lowest protein digestibility among the feedstuffs.
CONCLUSION
Supplementation of β-mannanase improved ME in barley, palm kernel meal, sorghum, and soybean meal, and ATTD of CP in copra meal and palm kernel meal for growing pigs. This study also indicates that various factors such as the structure and the amount of β-mannans, water binding capacity, and the level of resistant starch vary among feedstuffs and thus the efficacy of supplemental β-mannanase may be influenced by these factors.
Notes
CONFLICT OF INTEREST
We certify that there is no conflict of interest with any financial organization regarding the material discussed in the manuscript.
FUNDING
This study was financially supported from North Carolina Agricultural Foundation (#660101, Raleigh, NC, USA), USDA-NIFA (Hatch #02893), and CTCBIO Inc. (Seoul, Korea).
ACKNOWLEDGMENTS
The authors appreciate technical supports from Mr. Yunlong Shi, Dr. Alexandra C. Weaver, Dr. Yanbin Shen, Dr. Alysson Saraiva, Mr. Corey Ballou, and Ms. Gwendoline Voilque of Kim Lab at North Carolina State University (Raleigh, NC, USA).